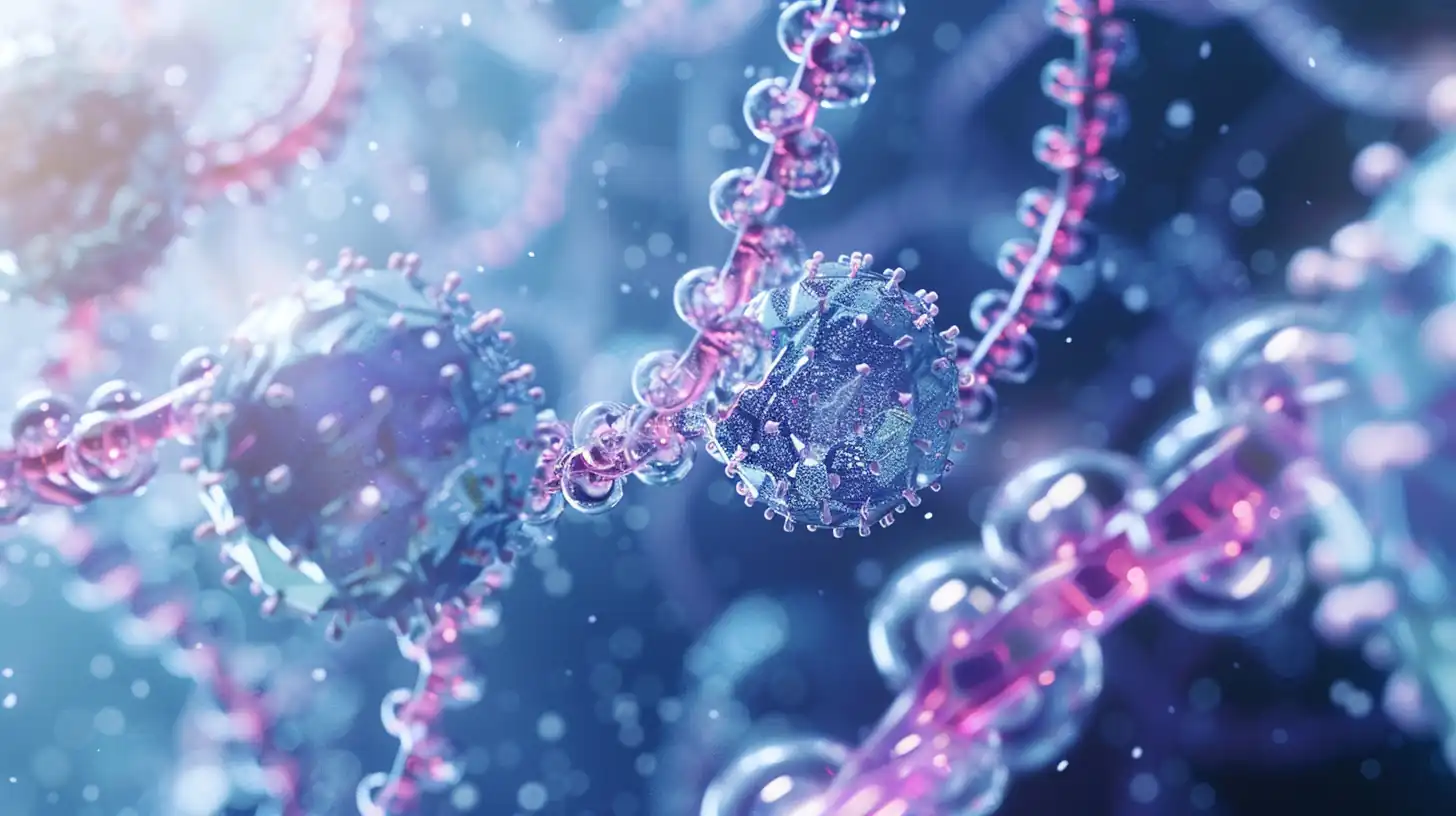
Somehow, nearly two meters of DNA fit inside a nucleus that’s only a few micrometers wide. Cells solve this with histone proteins. These small, positively charged proteins act like spools, helping DNA wrap around them in tight, organized loops. This structure keeps genetic material compact and prevents tangling.
In this study guide, we explain how histones form the nucleosome, how chemical changes to histones control access to DNA, and how DNA is packed during replication. You’ll also learn about chromatin structure, histone variants, and remodeling enzymes, with clear explanations and practical examples.
Histones: Quick Summary
Do you just need the basics? Here’s a simple explanation of what histones and chromatin structure are:
🟠 Histones are basic proteins rich in lysine and arginine that help package negatively charged DNA into compact chromatin structures.
🟠 A nucleosome consists of ~146 base pairs of DNA wrapped around a histone octamer made of H2A, H2B, H3, and H4.
🟠 The linker histone H1 stabilizes the DNA between nucleosomes and helps fold chromatin into a 30 nm fiber.
🟠 Histone modifications like acetylation and methylation change how tightly DNA is packed, influencing gene access.
🟠 ATP-dependent chromatin remodelers such as SWI/SNF reposition or remove nucleosomes to regulate DNA accessibility.
🟠 During DNA replication, histones are recycled or replaced by assembly factors like CAF-1, NAP-1, and Asf1 to maintain chromatin structure.
🟠 Nucleosome positioning shapes DNA accessibility, with transcription factors and remodelers creating open regions at active genes.
What is a Histone?
Histones are small, basic proteins found in the nucleus. They contain a high amount of lysine and arginine, two positively charged amino acids that attract the negatively charged DNA. This attraction allows histones to bind DNA tightly and compact it into an organized structure.
The first level of this organization is the nucleosome, where DNA wraps around a group of eight histones. These histones form a core known as the histone octamer. A separate histone, called the linker histone, attaches to the DNA between nucleosomes and stabilizes the structure.
Core histones:
- H2A
- H2B
- H3
- H4
Each nucleosome contains two copies of each of these.
Linker histone:
- H1
It binds to the DNA where it enters and exits the nucleosome and contributes to further folding of chromatin.
Histone Type | Copies per Nucleosome | Function | DNA Interaction Site |
H2A, H2B | 2 each | Core histone | DNA wrapped region |
H3, H4 | 2 each | Core histone | DNA wrapped region |
H1 | 1 | Linker histone | Linker DNA (entry/exit) |
How Histones Compact DNA into Chromatin
Histones organize long strands of DNA into compact units. This packaging starts with nucleosomes and continues through higher-order folding until DNA is dense enough to form chromosomes.
Each nucleosome forms when DNA wraps around a histone octamer. Under a microscope, nucleosomes look like beads on a string, with the “string” made of linker DNA. This is the 10 nm fiber, the first visible level of DNA folding.
These nucleosomes coil into the 30 nm fiber, a thicker structure that likely follows the solenoid model. In this model, nucleosomes twist into a spiral. The linker histone H1 binds at the entry and exit of DNA on each nucleosome and helps stabilize this fold.
The 30 nm fiber folds further into loops that attach to a scaffold. These loops allow the final levels of chromatin compaction, bringing the DNA into the form needed for mitosis. Together, these steps increase the packaging ratio, reducing DNA length by thousands of times.
Main levels of chromatin folding:
- 10 nm fiber – linear nucleosome chain
- 30 nm fiber – helical fiber with H1
- Loops and scaffolds – folded domains of chromatin
Structure of the Nucleosome
A nucleosome is made of 146 base pairs of DNA wrapped 1.67 times around a histone octamer. This octamer has two copies each of the histone proteins H2A, H2B, H3, and H4.
Histones use a structural motif called the histone fold, made of three alpha-helices separated by loops. These folds allow histones to form dimers and tetramers. First, H3 and H4 combine into a tetramer. Then, two H2A-H2B dimers bind on each side to complete the octamer.
DNA interacts with the octamer through many ionic and hydrogen bonds. These interactions are strong enough to keep the DNA in place but flexible enough to allow access when needed, such as during transcription or replication.
Histone Variants and Their Effects on Chromatin
Not all histones are identical. Some are slightly different versions called histone variants. These proteins replace standard histones in certain regions and change how chromatin behaves.
The most studied variants are found in H2A and H3. For example, H2A.Z can make chromatin more flexible, while macroH2A helps form compact structures in inactive regions. Another variant, H2AX, appears at DNA damage sites and signals for repair enzymes to act. In H3, the variant H3.3 appears in active genes, and CENP-A replaces H3 at centromeres to support chromosome segregation during cell division.
These changes don’t alter DNA but affect how tightly it is packed. Some variants loosen chromatin, making it easier for transcription to begin. Others help stabilize DNA in silent regions or during replication.
Histone variants are placed into nucleosomes by dedicated enzymes, often in response to cell signals. Because of them, not all nucleosomes are the same. This extra layer of control adds flexibility to how genes are accessed and regulated across different parts of the genome and in different cell states.
Histone Modifications and Chromatin Remodeling
Histones can change through small chemical reactions that affect how tightly DNA wraps around them. These changes help control which parts of DNA are open or closed for cellular processes.
Most modifications happen on the loose tail ends of histone proteins. They don’t change the DNA itself, but they influence how DNA interacts with proteins and how compact the chromatin is.
Types of histone modifications:
- Acetylation – added by histone acetyltransferases (HATs)
- Deacetylation – removed by histone deacetylases (HDACs)
- Methylation – added by specific methyltransferases
- Phosphorylation – often appears during cell division or DNA repair
- Ubiquitination – adds small proteins that change histone behavior
Acetylation reduces the positive charge on histones. This weakens their grip on DNA, loosening the structure. Deacetylation restores the charge and tightens the DNA wrapping. Methylation can either open or close the chromatin, depending on the location and number of methyl groups. Phosphorylation changes chromatin during mitosis, while ubiquitination can signal for structural shifts or histone removal.
These marks are reversible. Enzymes add and remove them in response to signals from the cell.
Common modification patterns include:
Activating Marks | Repressive Marks |
H3K9ac | H3K27me3 |
H3K4me3 | H3K9me3 |
Each mark signals a different chromatin state. You can think of these as labels that help organize the genome during transcription, replication, and repair.
ATP-Dependent Chromatin Remodeling
Cells also use protein machines to move histones on DNA. These machines are called chromatin remodeling complexes. They use energy from ATP to shift, eject, or reshape nucleosomes.
The most common complexes are SWI/SNF, ISWI, and CHD. Each works in a slightly different way, but they all change how tightly DNA wraps around histones.
These remodeling steps open or close access to parts of DNA. For example, a gene can stay inactive if histones block the start site. If a remodeling complex moves that nucleosome, transcription can begin.
This process happens constantly. It allows the cell to quickly respond to changes and keep different genes active in different places or at different times.
Histone Assembly and Recycling During DNA Replication
During DNA replication, histones are temporarily removed, then replaced to rebuild chromatin behind the replication fork. This keeps the DNA compact and organized.
H3 and H4 usually stay together as tetramers. These are split and passed randomly to both DNA strands. H2A and H2B are mostly replaced with new dimers.
Several proteins help with this process:
- CAF-1 places H3 and H4 on the new DNA
- NAP-1 loads H2A and H2B
- Asf1 holds fresh H3 and H4 before assembly
Old histones carry chemical marks that guide enzymes to modify new histones the same way.
Nucleosome Reassembly at the Replication Fork
Old histones are handed to daughter strands as the fork moves forward. New histones are added to replace any that were lost. This way, the chromatin structure is quickly restored. The same patterns of chemical tags are kept, so the new DNA strands match the original in both sequence and structure.
Nucleosome Positioning and DNA Accessibility
Nucleosomes sit at specific spots along DNA, and these positions affect how easily proteins can reach certain genes. Some sites stay open for transcription, while others are blocked by tightly packed histones.
The position of each nucleosome depends on several things:
- DNA sequence can attract or repel histones
- Histone variants can change how DNA is wrapped
- Remodeling enzymes move nucleosomes along the strand
- Transcription factors push histones aside to reach their target
At active promoters, you’ll often find nucleosome-free regions. These stretches give proteins full access to DNA, helping them begin transcription. The boundary nucleosomes near these regions are well-positioned and often marked with special histone variants.
Even when DNA is wrapped, it’s not always hidden. It unwraps for short periods—a process called DNA breathing. This brief exposure gives enzymes a chance to bind without removing the histone.
Useful markers and mechanisms:
- CTCF acts as a barrier and helps position neighboring nucleosomes
- Remodelers like SWI/SNF and INO80 shift or slide nucleosomes
- Frequent histone replacement at certain sites keeps DNA flexible for gene activity
This dynamic setup controls which genes are accessible and when.
Histone Chaperones and Nucleosome Stability
Histone chaperones help keep nucleosomes stable and guide histones to the right places during DNA packaging. These proteins bind histones to prevent them from forming random aggregates and ensure they are deposited properly.
Some chaperones act during transcription or repair, while others function during DNA replication. For example, FACT (Facilitates Chromatin Transcription) allows RNA polymerase to pass by temporarily disassembling and reassembling nucleosomes. Nucleoplasmin stores histones in oocytes and helps with chromatin assembly after fertilization.
Chaperones also help maintain the balance between free and bound histones, preventing stress in the nucleus. They can escort histones that carry specific marks, preserving epigenetic states across different stages of the cell cycle. Together with assembly factors like CAF-1 and NAP-1, histone chaperones make chromatin packaging efficient and controlled, especially when cells divide or respond to DNA damage.
Tutoring Support for Histones and Chromatin
Histones can get tricky. One minute you’re reading about DNA, and the next you’re trying to remember how many times it wraps around a histone octamer. Add modifications, ATP-remodeling, and replication to the mix, and it’s easy to get stuck. You don’t have to figure it out on your own.
With tutoring, you can go over chromatin structure at your pace. A private teacher helps break down things like H3K27me3, linker histones, or how CAF-1 works during replication. If you’re working through GCSE or A-level topics, it’s often easier to talk things through than read them alone.
You can find help near you—just search for “chemistry tutor Birmingham” or “tutoring chemistry Sheffield.” Online lessons with a real person mean you can ask anything, stop when needed, and go back over tough points.
If histone structure or chromatin remodeling feels like too much right now, that’s normal. Book a session on meet’n’learn with someone who gets it, and let’s work through it together. You’ll get through the details faster, and they’ll actually make sense.
Looking for more resources? Check out our Biology blogs for additional learning material. If you’re ready for extra help, a tutor can guide you through the most challenging topics with clarity and patience.
Histones: Frequently Asked Questions
1. What is a histone?
A histone is a basic protein that binds to DNA and helps organize it into chromatin.
2. What are the main types of histones?
The main histones are H2A, H2B, H3, H4 (core histones), and H1 (linker histone).
3. What is a nucleosome?
A nucleosome is a structure made of DNA wrapped around eight core histones.
4. How do histones interact with DNA?
Histones bind to negatively charged DNA through their positively charged amino acids, like lysine and arginine.
5. What is the function of the linker histone H1?
The linker histone H1 stabilizes DNA between nucleosomes and helps fold chromatin into higher-order structures.
6. What are common histone modifications?
Common histone modifications include acetylation, methylation, phosphorylation, and ubiquitination.
7. What is ATP-dependent chromatin remodeling?
ATP-dependent chromatin remodeling is the process of moving or restructuring nucleosomes using energy from ATP.
8. How does nucleosome positioning affect gene access?
Nucleosome positioning controls how easily enzymes and transcription factors reach DNA regions.
Sources:
1. Cell
2. Britannica
3. Wikipedia
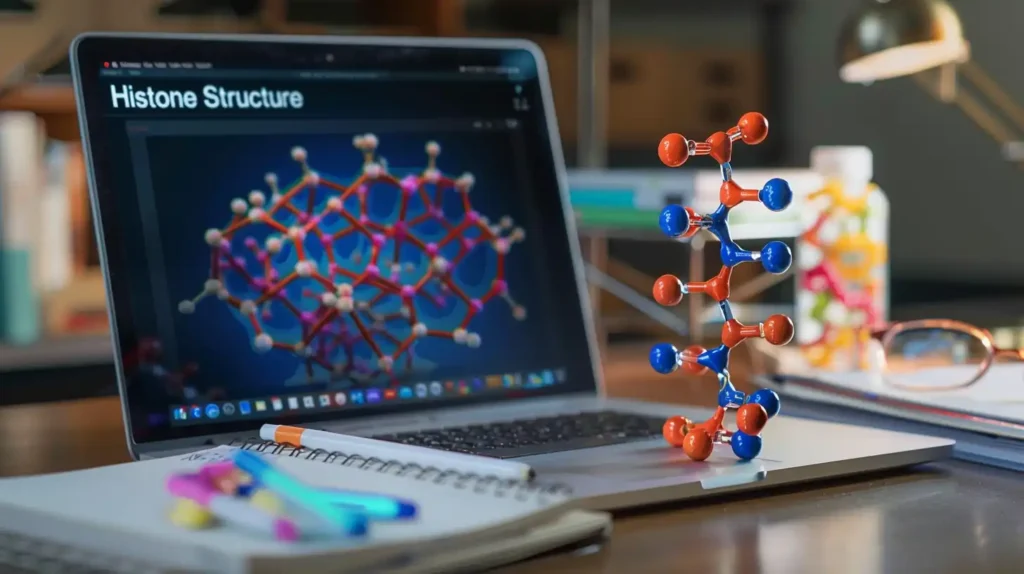