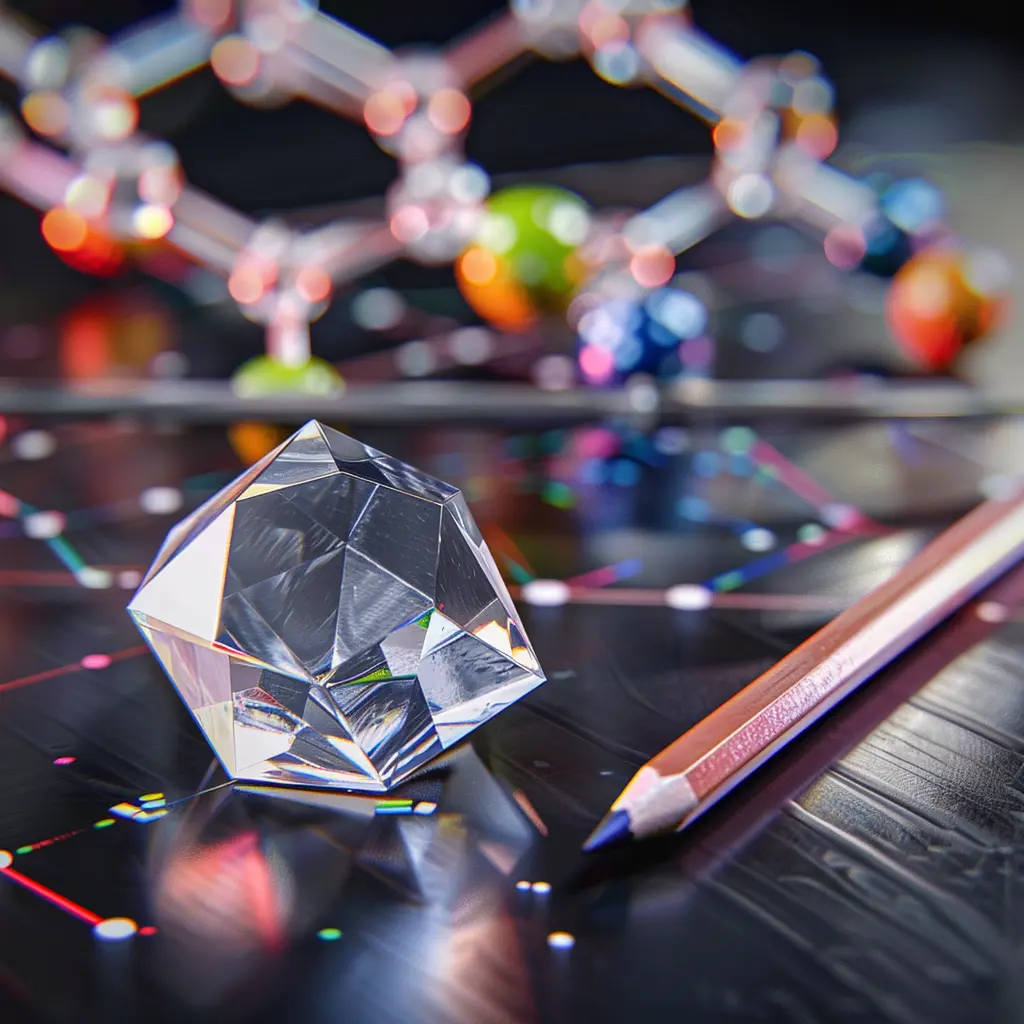
Imagine holding a diamond and a pencil. Both contain carbon, yet their properties are worlds apart.
This difference arises from allotropy, where elements exist in distinct forms in the same physical state. Allotropes like diamond and graphite showcase how atomic arrangements can drastically alter material characteristics.
In this study guide, we’ll explore allotropy, its definition, examples like allotropes of carbon and oxygen, and the conditions that influence their formation. You’ll learn how different structural forms of elements create variations in physical and chemical properties, with clear comparisons and explanations tailored for high school students.
Allotropy: Quick Summary
Do you just need the basics? Here’s a simple explanation of what is an allotrope:
🟠 Allotropy describes how elements like carbon or oxygen can exist in different structural forms within the same physical state.
🟠 Allotropes vary in atomic arrangements, creating differences in properties such as hardness, conductivity, or reactivity.
🟠 Conditions like temperature and pressure determine which allotrope is stable, with examples including tin transforming into a brittle form at low temperatures.
🟠 Polymorphism applies to compounds and explains how substances like calcium carbonate form different crystal structures depending on environmental factors.
What is Allotropy?
Allotropy describes the property of certain chemical elements to exist in two or more distinct structural forms within the same physical state. These forms, called allotropes, result from variations in atomic arrangements.
For example, carbon forms diamond and graphite, oxygen exists as O₂ and O₃, and phosphorus has white and red allotropes.
Key Features of Allotropy
- Found in elements in the same physical state.
- Caused by different atomic arrangements.
- Can vary with temperature, pressure, or light exposure.
Comparison of Allotropes
Element | Allotropes | Properties |
Carbon | Diamond, Graphite | Diamond: Hard, transparent; Graphite: Soft, flaky |
Oxygen | O₂, O₃ | O₂: Colorless, supports respiration; O₃: Blue, oxidizer |
Phosphorus | White, Red | White: Reactive, toxic; Red: Stable, safer |
Allotropy explains why the same element can look and behave so differently. By arranging atoms in unique ways, elements create materials with distinct properties, such as the hardness of diamond versus the softness of graphite.
Examples of Allotropes in Non-Metals
Non-metals form some of the most diverse allotropes, where the same element creates materials with completely different properties. These differences come from how atoms are arranged. Carbon, oxygen, and phosphorus are excellent examples, showing how allotropy can produce materials with unique behaviors and uses.
Allotropes of Carbon
Carbon has several well-known allotropes, each with unique structures. In diamonds, carbon atoms form a rigid tetrahedral lattice, making it extremely hard and transparent. Diamond doesn’t conduct electricity but transfers heat very effectively.
Graphite, on the other hand, has carbon atoms bonded in hexagonal layers. These layers slide over one another, making graphite soft and a good conductor of electricity. Graphene, a single layer of graphite, is one of the strongest materials known and is widely researched for its electrical conductivity and flexibility.
Fullerenes, including structures like buckyballs (C₆₀) and nanotubes, are closed shapes made of carbon atoms. These allotropes are used in advanced fields like nanotechnology due to their unique shapes and properties.
Allotropes of Oxygen and Phosphorus
Oxygen has two common allotropes. O₂ (dioxygen) is the form you breathe and is essential for life. It’s colorless and stable under normal conditions. O₃ (ozone) is a blue gas that acts as a strong oxidizer. It protects the Earth by absorbing harmful UV radiation in the atmosphere.
Phosphorus also exists in multiple forms. White phosphorus is made of P₄ molecules and is highly reactive, often stored under water to prevent ignition. Red phosphorus is more stable and safer to handle and is often found in safety matches. Black phosphorus has a layered structure like graphite and conducts electricity, making it valuable in electronics.
These examples show how changing atomic arrangements can lead to completely different materials, even within the same element.
Allotropes in Metals and Metalloids
Metals and metalloids also display allotropy, where variations in atomic arrangements create materials with distinct properties. While often less visually dramatic than non-metals, these allotropes are essential for understanding how these elements behave under different conditions. Examples include iron, tin, boron, silicon, and arsenic.
Allotropes in Metals
Many metals form allotropes that differ in structure based on temperature and pressure. Iron is a prime example. At room temperature, it exists as α-iron (ferrite) with a body-centered cubic structure. This form is magnetic and relatively soft. When heated to 912°C, iron transforms into γ-iron (austenite) with a face-centered cubic structure, which is non-magnetic and more malleable. Above 1,394°C, iron becomes δ-iron, reverting to a body-centered cubic arrangement but with different properties.
Tin also exhibits interesting allotropy. At normal temperatures, it forms β-tin (white tin), a metallic and stable form. However, when cooled below 13.2°C, it transforms into α-tin (gray tin), a brittle, non-metallic allotrope. This transformation, known as “tin pest,” is a unique phenomenon in metallurgy.
Allotropes in Metalloids
Metalloids, which have properties of both metals and non-metals, also show allotropy. Boron has multiple forms, including amorphous boron, which appears as a brown powder, and crystalline boron, which forms a hard, black solid. These structures differ in how boron atoms link into clusters.
Silicon forms amorphous and crystalline allotropes. Crystalline silicon, with its diamond-like structure, is a semiconductor widely used in electronics. Arsenic has allotropes, including gray arsenic, a metallic form, and yellow arsenic, which is molecular and less stable.
The allotropes of metals and metalloids highlight how atomic structures adapt to environmental conditions, producing materials with varying physical and chemical behaviors.
How Conditions Affect Allotropes
The form an element takes as an allotrope depends heavily on temperature, pressure, and light. Changes in these conditions rearrange the atoms in the element, often creating entirely different structures with unique properties.
Temperature can trigger dramatic changes. Tin pest is a well-known example where β-tin (white tin) transforms into α-tin (gray tin) at temperatures below 13.2°C. The brittle, non-metallic form causes tin objects to disintegrate in cold conditions. Iron, on the other hand, shifts from α-iron (ferrite) to γ-iron (austenite) at 912°C, becoming more malleable and ductile.
Pressure also plays a significant role. Carbon transforms into diamonds under high-pressure environments, while lower pressures favor graphite. Similarly, white phosphorus turns into black phosphorus when subjected to high pressure, adopting a stable, layered structure.
Light exposure can initiate slower changes. White phosphorus gradually converts into red phosphorus when exposed to light and heat over time, reducing its reactivity.
Each allotrope remains stable under specific conditions. When those conditions change, the atomic arrangement shifts, producing new forms with distinct physical and chemical behaviors. These transformations explain why elements like carbon or tin can exist in multiple forms, each suited to particular environments.
Allotropism vs. Polymorphism
Allotropism and polymorphism describe structural differences in substances but apply to distinct cases. Allotropism occurs in elements, where atoms bond differently to form unique structures in the same physical state. For instance, carbon forms diamond and graphite, while oxygen exists as O₂ and O₃.
Polymorphism applies to compounds where the same chemical formula produces different crystal structures. A common example is calcium carbonate (CaCO₃), which appears as calcite or aragonite depending on environmental conditions like temperature and pressure.
To compare, a diamond is an allotrope because it is a single element, carbon, arranged in a rigid tetrahedral structure. In contrast, silica (SiO₂) demonstrates polymorphism, forming different crystalline forms such as quartz, cristobalite, or tridymite.
Allotropism describes variations within elements, while polymorphism focuses on compounds. These differences explain how substances adapt to environmental changes and why their physical properties vary widely.
How Scientists Study Allotropy
Studying allotropy involves using advanced techniques to analyze atomic structures and observe how they change. X-ray crystallography is one of the most important tools. It allows scientists to map out the precise arrangement of atoms in solids, helping to reveal the differences between structures like diamond and graphite.
Spectroscopy is another essential method for studying changes in molecular structures. Researchers use this technique to track transformations, such as how white phosphorus converts into red phosphorus under heat or light. These observations show how allotropes form and remain stable under specific conditions.
High-pressure experiments simulate environments deep within Earth’s crust, where elements like carbon can transition from graphite to diamond. These controlled conditions allow scientists to study the effects of pressure on atomic arrangements and explore the creation of new materials.
Electron microscopy provides high-resolution images of atomic arrangements. This technology is particularly useful for newer allotropes like graphene, where scientists need to observe individual layers of atoms.
These methods help researchers explore the behavior of elements and understand how changes in temperature, pressure, or light influence their allotropes. By using these tools, scientists uncover how atomic structures shape the properties of materials we use every day.
Advance Your Knowledge in Allotropy with a Tutor
Chemistry sometimes feels overwhelming, especially when working through challenging topics or preparing for exams. If you’re stuck on a concept, finding the right support can make your learning process much smoother and more effective.
A tutor provides personalized attention, helping you focus on areas you need the most improvement. One-on-one tutoring lets you ask questions and move at your own pace, ensuring you fully grasp the material. With tailored lessons, you can confidently approach difficult topics and make steady progress.
Chemistry classes can be a great option if you enjoy learning with others. Group lessons encourage discussion, offer new perspectives, and often include hands-on activities to deepen understanding.
You can find more useful topics in our Chemistry blogs. If you’re looking for extra help, a tutor can guide you through challenging concepts in a way that makes sense.
Search for a tutor using phrases like “chemistry tutor Newcastle” or “chemistry teacher Sheffield” on platforms like meet’n’learn. You’ll find someone who can tailor lessons to your needs.
If you prefer learning in a group, search for “chemistry classes Nottingham” or “chemistry lessons Oxford” online. These searches will lead you to chemistry tutoring options nearby.
Allotropy: Frequently Asked Questions
1. What is allotropy?
Allotropy describes how an element can exist in different structural forms within the same physical state. These forms, called allotropes, result from variations in atomic arrangements and bonding.
2. What are some examples of allotropes of carbon?
Carbon forms allotropes like diamond, graphite, graphene, and fullerenes. Diamond has a rigid, hard structure, while graphite is soft and conductive due to its layered arrangement.
3. How does temperature affect allotropes?
Temperature changes can trigger transitions between allotropes. For example, tin transforms from metallic β-tin to brittle α-tin at temperatures below 13.2°C.
4. How is allotropy different from polymorphism?
Allotropy applies to elements, while polymorphism describes how compounds like calcium carbonate can form different crystal structures, such as calcite and aragonite.
5. Can all elements exhibit allotropy?
No, only certain elements like carbon, oxygen, and phosphorus have allotropes. Many elements maintain the same structure under varying conditions.
6. Why do allotropes have different properties?
Allotropes have different properties because their atoms are bonded in distinct ways. For instance, diamond’s structure makes it extremely hard, while graphite’s layers make it soft and slippery.
7. What are some common allotropes of phosphorus?
Phosphorus exists in forms like white phosphorus, which is reactive; red phosphorus, which is stable; and black phosphorus, which has a layered and conductive structure.
8. Can pressure influence allotropy?
Yes, pressure can alter allotropes. For example, high pressure turns graphite into diamond and white phosphorus changes into black phosphorus under similar conditions.
Sources:
1. ThoughtCo
2. Britannica
3. Wikipedia
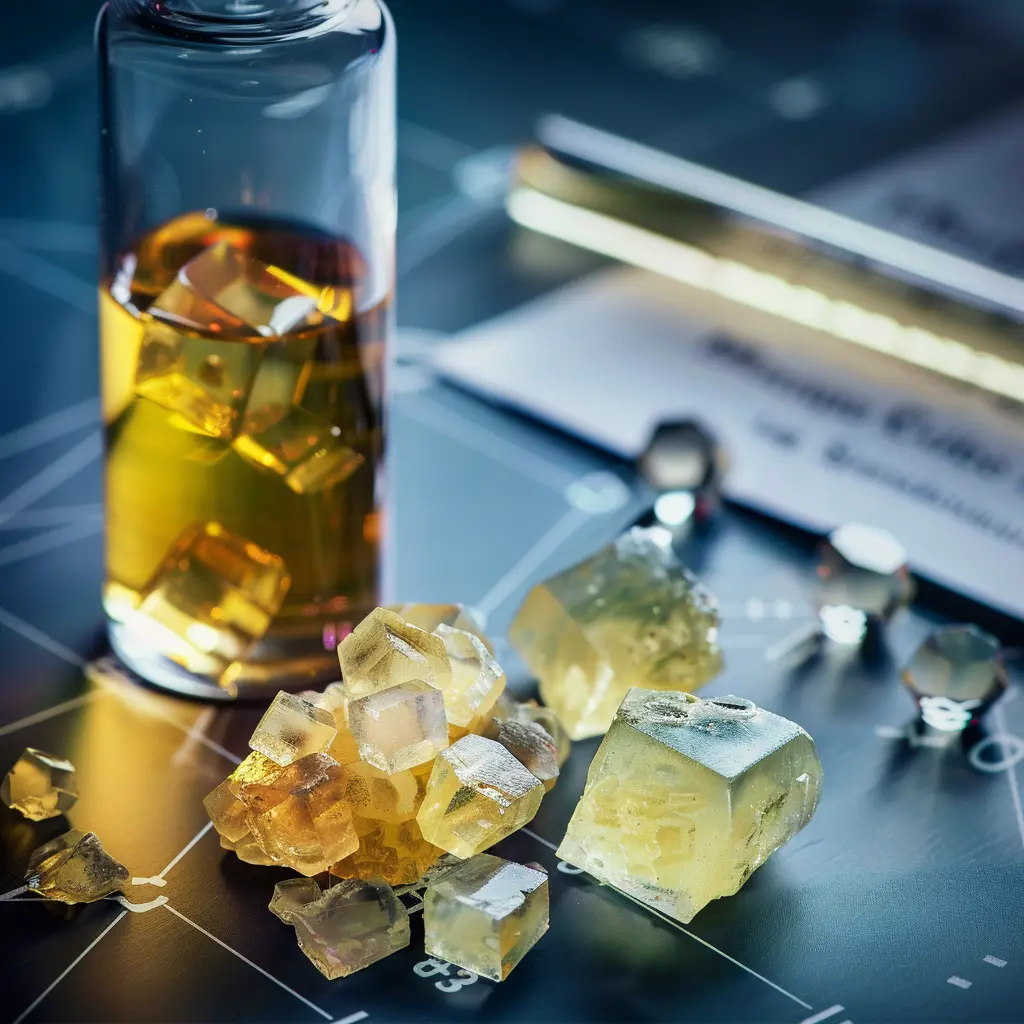