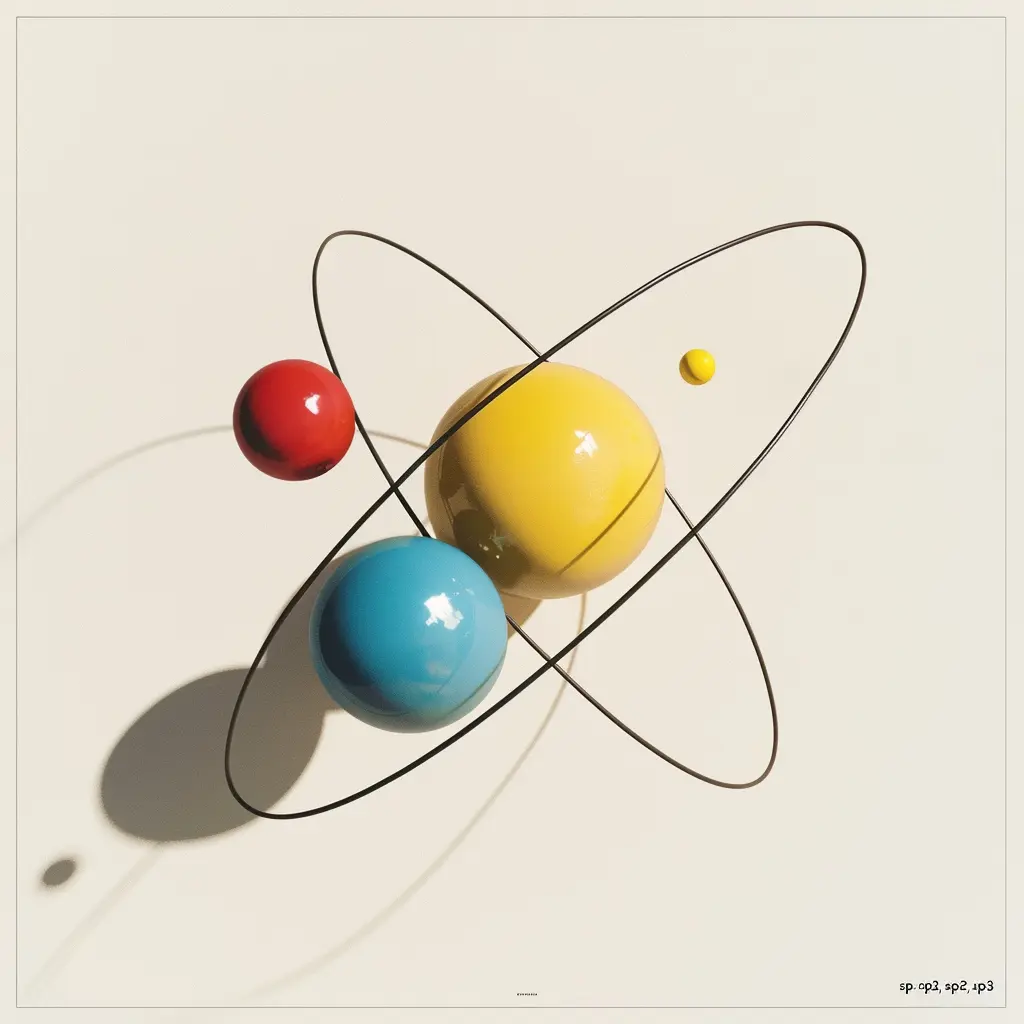
When carbon dioxide ($CO_2$) forms its linear shape or methane ($CH_4$) adopts a tetrahedral structure, the explanation is hybridization.
Hybridization combines atomic orbitals into arranged hybrid orbitals to maximize bonding efficiency and minimize electron repulsion.
We will explain hybridization step-by-step. You’ll learn how to identify the type of hybridization (sp, sp², sp³), connect it to molecular geometry, and analyze examples like $CH_4$, $C_2H_4$, and $CO_2$.
Hybridization: Quick Summary
Do you just need the basics? Here’s a simple explanation of hybridization and hybrid orbitals:
🟠 Hybridization combines atomic orbitals to form new orbitals used in bonding.
🟠 sp Hybridization occurs when one s and one p orbital mix, resulting in a linear shape with 180° bond angles.
🟠 sp² Hybridization involves one s and two p orbitals, leading to a trigonal planar structure with 120° bond angles.
🟠 sp³ Hybridization forms from one s and three p orbitals, creating a tetrahedral geometry with 109.5° bond angles.
🟠 Hybrid orbitals form by combining atomic orbitals to create new shapes, enabling specific molecular geometries like tetrahedral, trigonal planar, or linear structures.
What Is Hybridization?
Hybridization combines atomic orbitals to form new hybrid orbitals better suited for bonding. These hybrid orbitals influence molecular shapes and bond angles, often not explained by individual atomic orbitals alone.
For example, in methane ($CH_4$), carbon’s single $s$ orbital and three $p$ orbitals mix to create four equivalent sp³ hybrid orbitals. These orbitals point toward the corners of a tetrahedron, giving methane its characteristic shape with bond angles of 109.5°.
Comparing Hybridization Types
Hybridization | Orbitals Involved | Geometry | Example | Bond Angle |
sp | 1 s, 1 p | Linear | $CO_2$, $C_2H_2$ | 180° |
sp² | 1 s, 2 p | Trigonal planar | $BF_3$, $C_2H_4$ | 120° |
sp³ | 1 s, 3 p | Tetrahedral | $CH_4$, $NH_3$ | 109.5° |
Hybridization also helps explain why some molecules form double or triple bonds. For example, in ethene ($C_2H_4$), each carbon atom forms sp² hybrid orbitals for single bonds while keeping an unhybridized $p$ orbital for the double bond.
Types of Hybridization
Hybridization explains the combination of atomic orbitals to form hybrid orbitals with specific geometries. These geometries influence the shapes of molecules and their bond angles. The type of hybridization depends on the number of atomic orbitals involved and the bonding structure.
sp Hybridization
In sp hybridization, one $s$ orbital and one $p$ orbital combine to form two equivalent sp hybrid orbitals. This hybridization occurs in molecules with a linear geometry, where atoms align in a straight line.
The bond angle in sp hybridized molecules is 180°, ensuring minimal repulsion between bonded atoms. A typical example is carbon dioxide ($CO_2$), where the central carbon atom uses sp hybrid orbitals to form double bonds with oxygen. Another example is ethyne ($C_2H_2$), where carbon atoms form a triple bond.
sp² Hybridization
In sp² hybridization, one $s$ orbital and two $p$ orbitals combine to form three sp² hybrid orbitals. These orbitals are arranged in a trigonal planar geometry, where atoms lie in a single plane with bond angles 120°.
This hybridization is observed in molecules like boron trifluoride ($BF_3$), where the central boron atom forms three equivalent bonds. In ethene ($C_2H_4$), each carbon atom uses sp² hybrid orbitals to form single bonds with hydrogen and a double bond with another carbon atom.
sp³ Hybridization
In sp³ hybridization, one $s$ orbital and three $p$ orbitals combine to form four equivalent sp³ hybrid orbitals. These orbitals arrange themselves in a tetrahedral geometry, with bond angles of 109.5° to minimize electron pair repulsion.
Methane ($CH_4$) is a classic example, where the central carbon atom forms four single bonds with hydrogen. In ammonia ($NH_3$), the nitrogen atom adopts sp³ hybridization, with one lone pair and three bonds creating a slightly distorted tetrahedral shape.
sp³d Hybridization
In sp³d hybridization, one $s$ orbital, three $p$ orbitals, and one $d$ orbital mix to form five sp³d hybrid orbitals. These orbitals create a trigonal bipyramidal geometry with two distinct bond angles: 120° for bonds in the equatorial plane and 90° for bonds between equatorial and axial positions.
A typical example is phosphorus pentafluoride ($PF_5$), where the phosphorus atom bonds to five fluorine atoms. This geometry allows for five bonding regions, letting elements like phosphorus exceed the octet rule using empty $d$ orbitals.
sp³d² Hybridization
In sp³d² hybridization, one $s$ orbital, three $p$ orbitals, and two $d$ orbitals combine to form six sp³d² hybrid orbitals. These orbitals arrange themselves in an octahedral geometry, where all six bonding regions are evenly spaced at 90° angles.
An example is sulfur hexafluoride ($SF_6$), where the sulfur atom bonds symmetrically to six fluorine atoms. This arrangement minimizes repulsion and explains how elements like sulfur can expand their valence shells to bond with more than four groups.
These expanded hybridizations are common in elements from the third period and beyond, where $d$ orbitals become available for bonding.
Hybridisation Of The C Atom
Carbon’s ability to form sp, sp², and sp³ hybrid orbitals explains its versatility in bonding. In sp hybridization, carbon creates two linear orbitals with 180° angles, as seen in $C_2H_2$. In sp² hybridization, one $s$ and two $p$ orbitals combine, forming trigonal planar shapes with 120° angles, like in $C_2H_4$.
In sp³ hybridization, one $s$ and three $p$ orbitals produce tetrahedral geometries with 109.5° angles, as in $CH_4$. These hybridizations allow carbon to create stable single, double, and triple bonds, forming the backbone of countless organic molecules.
Hybrid Orbitals
Hybrid orbitals form during hybridization when atomic orbitals combine, creating new orbitals that determine molecular geometries and bond angles. These orbitals have directional properties, maximizing overlap with other orbitals for stronger covalent bonds.
For example, sp orbitals result in linear structures with 180° bond angles, as in $CO_2$. Sp² orbitals form trigonal planar geometries with 120° angles, like in $C_2H_4$, while sp³ orbitals arrange atoms in a tetrahedral shape with 109.5° angles, as in $CH_4$.
In double bonds, sp² orbitals form sigma bonds, while unhybridized $p$ orbitals create pi bonds, such as in ethene ($C_2H_4$). Like in acetylene ($C_2H_2$), triple bonds involve sp orbitals for sigma bonds, with two unhybridized $p$ orbitals forming the pi bonds.
How to Determine Hybridization
Determining hybridization involves a few straightforward steps based on molecular structure and electron regions. Following this method, you can quickly identify most molecules’ hybridization types.
1. Draw the Lewis Structure
Start by sketching the Lewis structure of the molecule to visualize bonded atoms and lone pairs of electrons around the central atom. For example, in $CH_4$, carbon bonds to four hydrogen atoms.
2. Count the Steric Number
The steric number is the sum of bonded atoms and lone pairs around the central atom.
- For $CH_4$, carbon has four bonded atoms and no lone pairs, so the steric number is 4.
- For $C_2H_4$, each carbon atom has three bonded atoms and no lone pairs, giving a steric number of 3.
- For $CO_2$, carbon has two bonded atoms and no lone pairs, resulting in a steric number of 2.
3. Match the Steric Number to Hybridization
- Steric Number 2 → sp hybridization (linear geometry, 180° bond angle).
- Steric Number 3 → sp² hybridization (trigonal planar geometry, 120° bond angle).
- Steric Number 4 → sp³ hybridization (tetrahedral geometry, 109.5° bond angle).
4. Address Exceptions
Some molecules deviate from typical patterns. For example:
- $PF_5$ has a steric number of 5, resulting in sp³d hybridization (trigonal bipyramidal geometry).
- $SF_6$ has a steric number 6, corresponding to sp³d² hybridization (octahedral geometry).
This method applies to most molecules and covers standard hybridization types while accounting for exceptions in expanded valence shells.
Hybridization and Molecular Geometry
Hybridization directly influences molecular geometry by determining how orbitals mix and arrange themselves in space. The resulting shapes align with the Valence Shell Electron Pair Repulsion (VSEPR) theory, which states that electron pairs around a central atom repel each other to maximize distance.
Electron Repulsion and Bond Angles
Hybridized orbitals form specific geometries to minimize repulsion between bonding and non-bonding electron pairs. The bond angles are a result of this arrangement:
sp hybridization: Linear geometry arises when two hybrid orbitals align 180° apart, minimizing repulsion. Example: In $C_2H_2$ (acetylene), the carbon atoms form a linear molecule.
sp² hybridization: Trigonal planar geometry occurs when three hybrid orbitals arrange themselves 120° apart in a flat plane. Example: In $BF_3$, boron forms a planar molecule with fluorine atoms.
sp³ hybridization: Tetrahedral geometry results when four hybrid orbitals are 109.5° apart in 3D space. Example: In $CH_4$ (methane), carbon forms a tetrahedron with hydrogen atoms.
VSEPR and Molecular Shapes
Hybridization provides a framework for predicting molecular geometry, while VSEPR theory explains why orbitals adopt specific arrangements. Together, they allow chemists to understand how atoms bond and how molecules take on their shapes.
Common Misconceptions About Hybridization
Hybridization is a fundamental concept in chemistry, but it’s often misunderstood. Below are some common misconceptions clarified:
1. Hybridization applies only to covalent bonding
It doesn’t explain the behavior of ionic compounds like $NaCl$ because these don’t share electrons to form bonds.
2. It doesn’t cover all molecular interactions
Hybridization focuses on atomic orbital mixing in covalent bonds. For example, it doesn’t account for weak intermolecular forces like hydrogen bonding or Van der Waals forces.
3. Hybridization doesn’t “change” during reactions
Once hybrid orbitals form, they remain constant unless a new bonding scenario arises. For instance, in $CH_4$, the $sp^3$ hybridization doesn’t alter as long as methane remains intact.
Advance Your Knowledge of Hybridization
Are you struggling with hybridization and hybrid orbitals? You can find more useful topics in our Chemistry blogs. Or find a tutor who can explain it clearly and simply.
Search for a tutor using phrases like “chemistry tutor Edinburgh” or “chemistry teacher Liverpool” on platforms like meet’n’learn. You’ll find someone who can tailor lessons to your needs.
If you prefer learning in a group, search for “chemistry classes Leeds” or “chemistry lessons London” online. The search will lead you to chemistry tutoring nearby.
Hybridization: Frequently Asked Questions
1. What is hybridization in chemistry?
Hybridization combines atomic orbitals (e.g., $s$, $p$) to form hybrid orbitals that facilitate molecule bonding.
2. How do you determine the hybridization of an atom?
To determine hybridization, draw the Lewis structure, count the steric number (bonded atoms + lone pairs), and match it to a hybridization type.
3. What is sp hybridization?
In sp hybridization, one $s$ orbital and one $p$ orbital combine to form two hybrid orbitals, resulting in a linear geometry with 180° bond angles.
4. How does sp² hybridization work?
Sp² hybridization combines one $s$ orbital and two $p$ orbitals to create three hybrid orbitals, forming a trigonal planar geometry with 120° bond angles.
5. What is sp³ hybridization?
Sp³ hybridization involves mixing one $s$ orbital and three $p$ orbitals to create four hybrid orbitals with a tetrahedral geometry and 109.5° bond angles.
6. Does hybridization explain ionic compounds?
No, hybridization only describes covalent bonding and does not apply to ionic compounds like $NaCl$.
7. Can hybridization change during a chemical reaction?
Hybridization typically remains constant unless a new molecular structure forms, such as when $BF_3$ reacts to form $BF_4^-$.
8. How does hybridization relate to molecular geometry?
Hybridization determines the geometry of a molecule by defining the arrangement of electron pairs around the central atom, as predicted by VSEPR theory.
Sources:
1. LibreTexts Chemistry
2. Geeks for Geeks
3. Wikipedia
